Yan Lab Research
Mission Statement
-
Explore complex and dynamic self-assembly using DNA as programmable molecules.
-
Use DNA self-assembly for the finest positional control of molecules for the construction of interactive spatial networks of chemical and biochemical species.
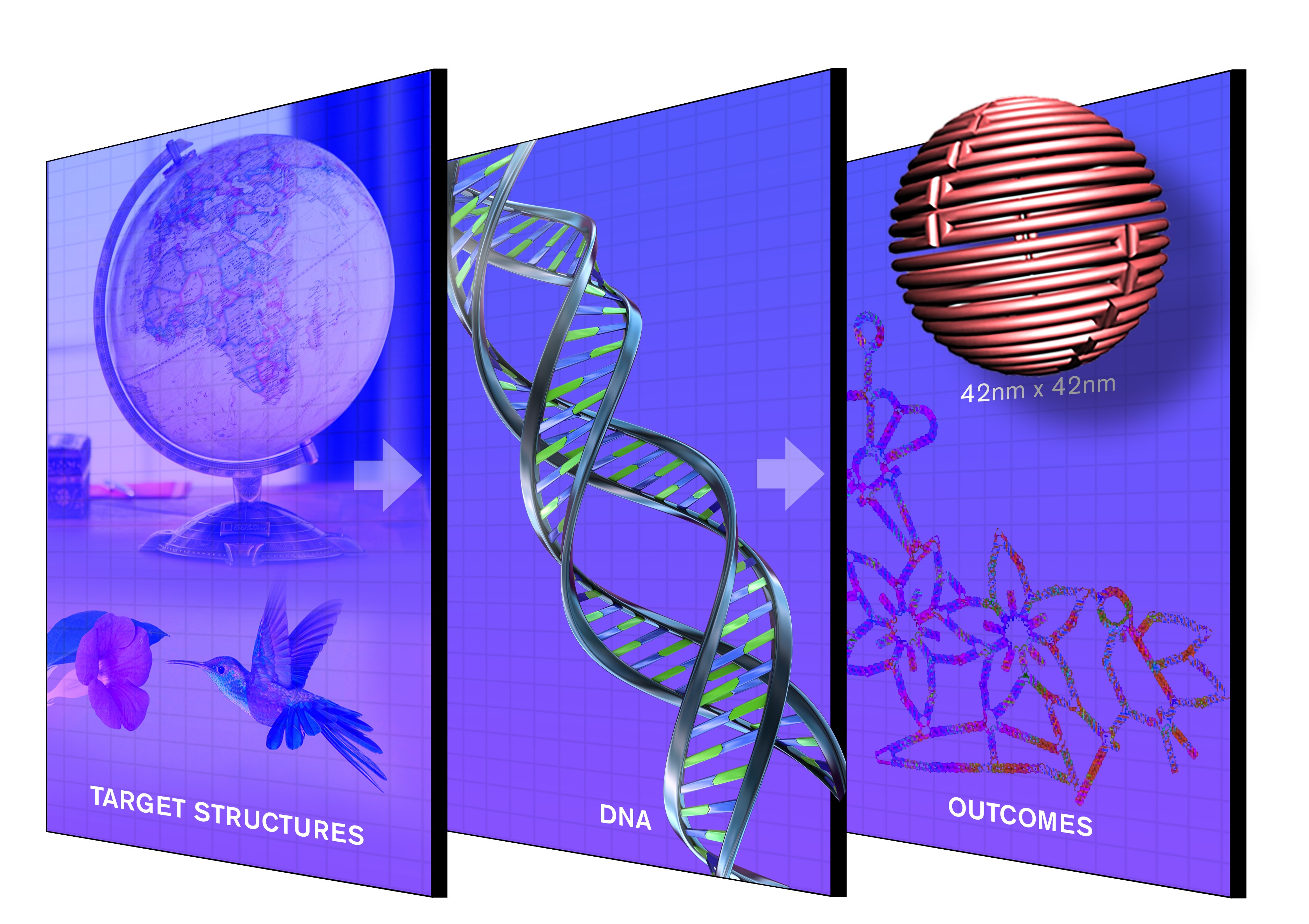
Design: Self-assembly is a remarkable process that Nature uses to organize chemical systems composed of non-living components into living, biological systems. Nature accomplishes this incredible feat by adding information to matter and by guiding the self-assembly process to create functional structures. DNA, nature's molecule of choice for storing and transmitting genetic information, is an excellent nanoscale building block for the construction of designer nano-architectures because of its specific three-dimensional (3D) conformation, chemical addressability, and predictable Watson-Crick base pairing. The most significant underlying concept in structural DNA nanotechnology is the application of immobile, branched DNA junctions, together with sequence specific sticky end associations, to create self-assembling arrays, objects and devices. DNA nanostructures exhibiting significant geometric and topological complexity can be designed conveniently using user-friendly software interfaces that have been developed (including several from our own lab) in the field of structural DNA nanotechnology. Both bottom-up and top-down design strategies have been developed to identify appropriate DNA oligonucleotide sequences that can fold and assemble into the target structures and their higher order assemblies through proper molecular interactions.
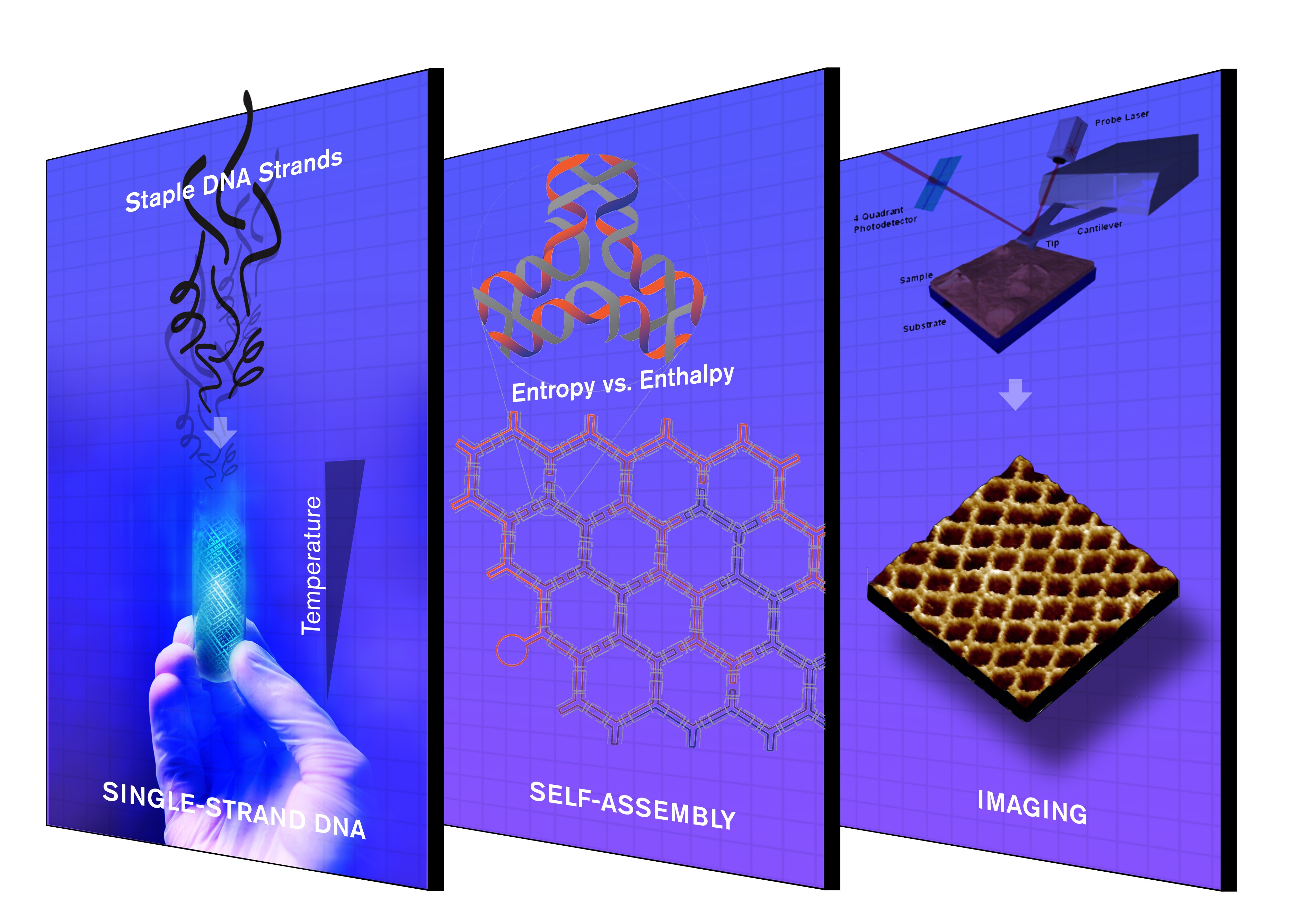
Assembly: Investigating how individual molecular components interact within DNA nanoarchitectures, both in terms of spatial and temporal interactions, is fundamental for understanding their resulting physical behaviors. This information will provide valuable insight to guide the design of more complex higher-order structures that can assemble more efficiently. Our lab has made important progress investigating the thermodynamics and kinetics of DNA structures with increasing complexity, which offer guidelines for both structural and computational purposes. We are interested in applying what we have learned from these fundamental studies to not only improve the DNA self-assembly and folding processes, but also design and engineer DNA nanostructures to achieve highly programmable hierarchical, dynamic, algorithmic, developmental and scalable self-assembly systems with finer spatio-temporal controls.
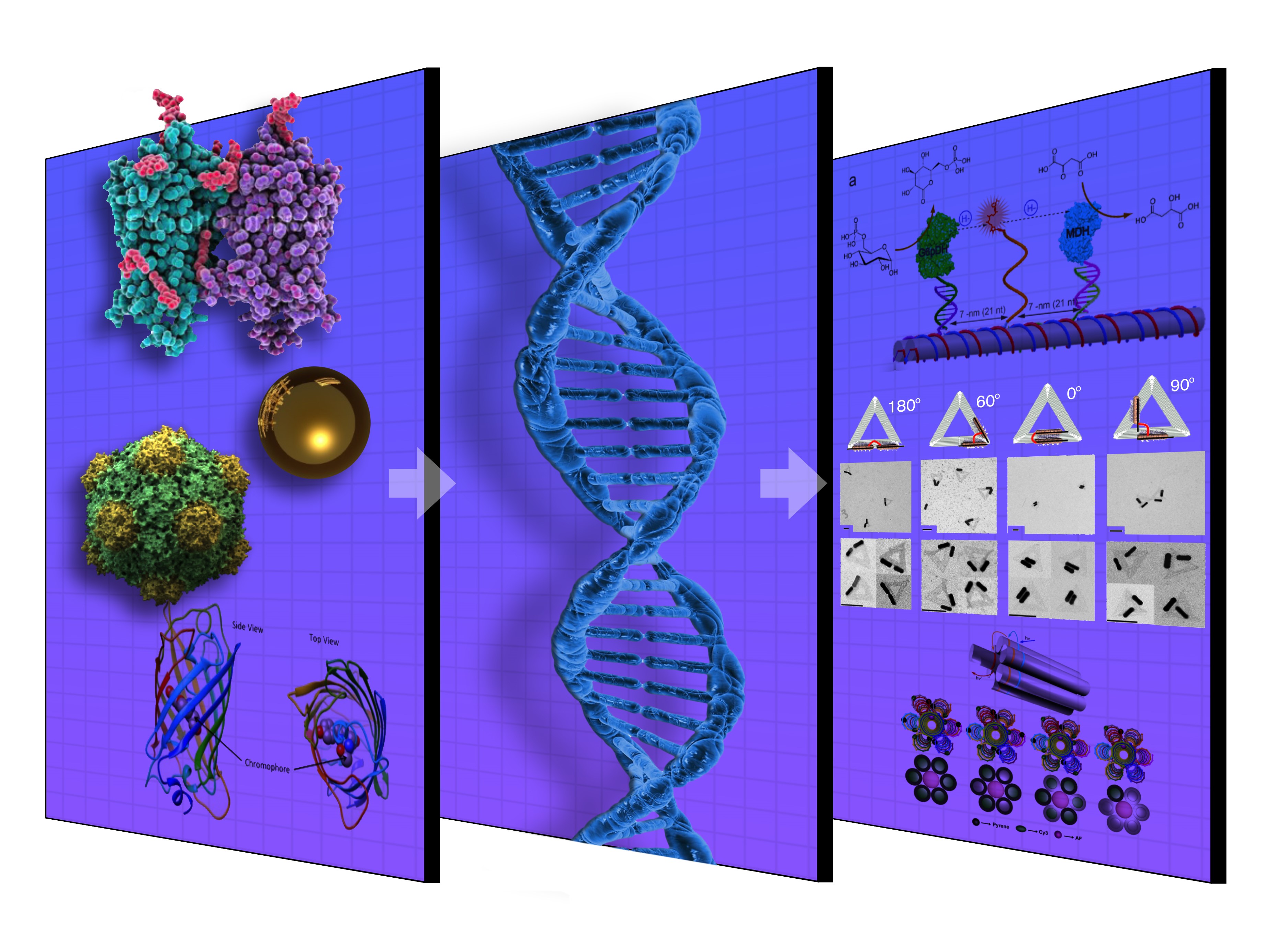
Functionalization: Taking advantage of the chemical addressability of DNA nanostructures, one can use DNA as molecular scaffolds to control the assembly of biomolecules and inorganic materials and probe their interactions. By exploiting the programmability of DNA nanostructures to accurately template functional molecules, materials and probes, we are able to organize these external elements into practical devices and engineer molecular sensors, circuits, and actuators. Over the years, we have successfully used rationally designed DNA nanoscaffolds to organize metallic nanoparticles, semiconductor nanocrystals into well-defined architectures. These inorganic particle-DNA nanostructure complexes have enabled systematic investigation of distance dependent interactions between photonic elements. In addition, we have used DNA nanostructures to organize various organic chromophores into artificial light harvesting complexes with control over cascading, unidirectional energy transfer. We have also used DNA nanostructures as chaperones to align and organize protein molecules using different bioconjugation strategies to engineer enzyme cascades and study protein-protein interactions. These developments pave the way for potential functional nanoscale and molecular scale devices with sense-compute-actuate elements built into the system.
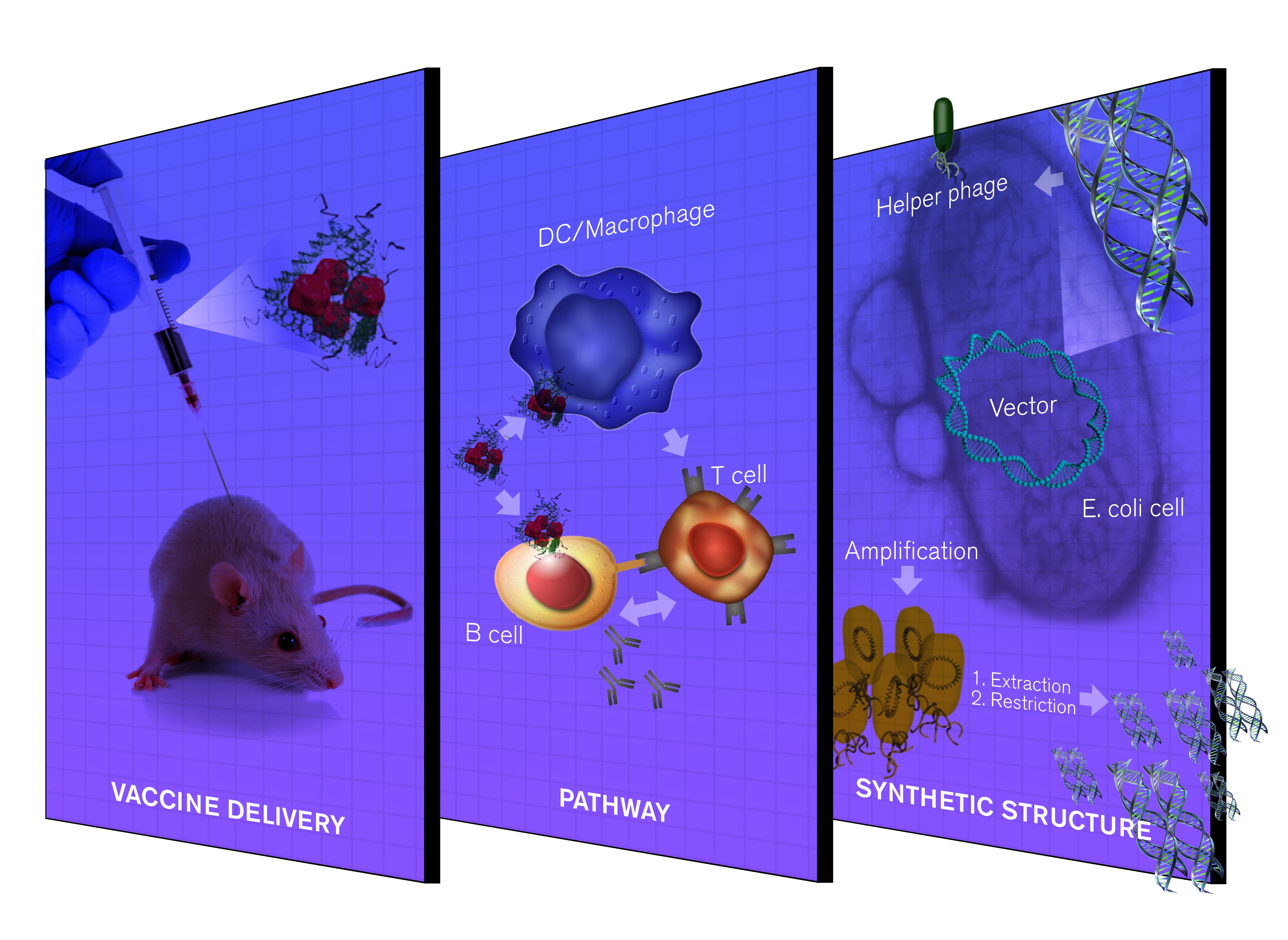
Interface: Structural DNA nanotechnology has emerged as a useful tool for both biomedical and material research applications. The intrinsic biocompatibility, nanoscale dimensions, programmability, and ability for functionalization of DNA nanostructures make them ideal platform to interface with cellular and in vivo systems. DNA nanostructures are promising vehicles for targeted drug delivery and have been used as synthetic vaccine platforms. DNA based molecular robotics can be designed to dynamically interact with each other and perform logic computations in vivo, opening up opportunities to develop smart theranostic nanodevices. We are interested in how DNA nanostructures interact with biological structures in vitro and in vivo, dissecting their intracellular behaviors and transport mechanisms, as well as functionalizing DNA nanostructures to improve their in vivo targeting and delivery properties. DNA nanostructures have also shown great success in directing the assembly of nanoelectronic and photonic elements. We are interested in developing a programmable chemical interface using DNA directed assembly to bridge self-assembling biomaterials and functional inorganic materials.